The world's oldest gorilla fossil has been found in Ethiopia, defying earlier assumptions about ape and human evolution, scientists at the National Museum of Ethiopia announced Wednesday.
Scientists believe the nine teeth unearthed during an excavation near Addis Ababa belong to a newly discovered type of 10-million-year-old gorilla. If their data is correct, the fossil could reveal several new truths on ape and human evolution.
"We used to believe, based on genetic information, DNA studies and molecular studies, that the splits between chimpanzees and the human line on one side and the gorilla line on the other side … happened around eight million years ago," said paleontologist Berhane Asfaw, who helped unearth the fossil. "But based on this new information, the split had to happen before 10 million years ago. It means that information has to be adjusted in every textbook."
The new species — dubbed Chororapithecus abyssinicus, a combination of the names Chorora, the area where the fossil was found, and Abyssinia, Ethiopia's ancient name — could indicate that the closely related gorillas and chimpanzees diverged as long as 12 million years ago, four million years earlier than previously thought.
Prior to the find, it was also thought that apes originated in Asia. Before Wednesday, the oldest known ape fossils were eight-million-year-old remains found in Pakistan and China. Previously, no gorillas had been found north of Kenya.
The fossil also represents the first discovery of a great ape in Ethiopia.
However, Ethiopia is home to one of the world's best-known fossil finds. The 3.5-million-year-old Australopithecus afarensis, better known as Lucy, which was discovered in Ethiopia in 1974, is widely acknowledged as one of man's earliest ancestors and one of the most complete ancient fossils ever found.
Since they found only teeth, scientists could not say what this newly discovered gorilla might have looked like. However, paleontologists said they were preparing to embark on an expedition to the region to seek more information.
Their findings will be published in Thursday's edition of the scientific journal Nature.
With files from the Associated Press
This is a pretty good chart which avoids drawing the lines of descent, from same site as the chart above it. The two small forms at far right would be perfect matches for the corresponding modern reports with the addition of bushy head-hair to go along with their hairy bodies.
1) Chaire de Paléoanthropologie et de
Préhistoire du Collège de France 2) Département Histoire de la Terre du Muséum
National d’Histoire Naturelle
This paper concerns four ape-like teeth from Kenya, one from the Ngorora
Formation (12.5 Ma), and three from the Lukeino Formation (5.9 Ma), collected by
the Kenya Palaeontology Expedition.
In this paper we restrict the term ‘hominid’ to encompass only those
hominoids that possess skeletal morphology indicative of habitual or obligate
bipedal locomotion. We do not employ it in the much wider sense that has
recently been used by some authors, in which even the genera
Pan and
Gorilla are included in the family Hominidae.
Bar 91’99 is an unworn right lower molar, probably the second (11.4 mm
mesiodistal × 9.4 mm buccolingual), although a case could be made for it being a
third molar. The apices of the protoconid and hypoconid are buccolingually
compressed and not very voluminous. They are located towards the buccal margin
of the tooth and they express minor buccal flare. The apices of the protoconid
and metaconid are 5.2 mm apart and the tooth is 9.2 mm broad at this level. The
protoconid and metaconid have low-relief, centrally directed crests which close
off a low walled mesial fovea. Anterior crests from these same cusps meet
mesially to wall off the anterior side of the mesial fovea, which is
buccolingually wide and mesiodistally short. The hypoconid has low crests
descending from its apex mesially and distally but not crossing the grooves,
which separate the cusp from its neighbors. There is a basal cingular enamel
fold between the protoconid and hypoconid and a tiny fold between the hypoconid
and hypoconulid. The hypoconulid has a crest descending obliquely anteriorly
into the talonid basin, but it is low and does not separate the basin from the
distal fovea. The postcristid reaches lingually towards the low cusplet present
between the hypoconulid and the entoconid.
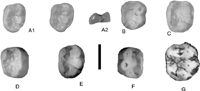
View Details |
Figure 1.
Selected hominoid
teeth from Africa and Europe (scale bar, 10 mm). (A) Bar 91’99, right lower
molar (cast); (A1) stereo occlusal view; (A2) mesial view to show buccal flare.
(B) Pan paniscus right m/2, occlusal view. (C) Pan troglodytes,
cast of right m/2, occlusal view. (D–F) Dryopithecus brancoi casts of
lower molars from Europe, occlusal views (D, Trochtelfingen; E, Salmendingen; F,
Ebingen). (G) Gorilla gorilla lower m/2, occlusal
view. |
The apices of the metaconid and entoconid are strongly buccolingually
compressed and peripherally positioned, a disposition that results in a
voluminous sunken talonid basin bordered by cusps that are somewhat trenchant in
appearance. The posterior crest of the metaconid descends towards a low,
peripherally positioned cusplet between the metaconid and entoconid. The
hypoconid extends beyond the midline of the crown and reaches this small
cusplet. As a consequence, the metaconid and entoconid do not touch each other.
A postentoconid crest descends obliquely towards another low cusplet located
between the entoconid and hypoconulid from which it is separated by narrow but
short vertical grooves on the posterolingual surface.
The occlusal basin is wide, deep, and long. The wall between the anterior
fovea and the talonid basin is low and the posterior fovea is not clearly
demarcated from it, and thus extends for almost the entire length of the tooth.
The occlusal surface of the enamel in the basin is wrinkled.
The main differences from molars of
Pan paniscus (
Figure 1B) concern the buccal cusps, which are slightly more
internally positioned in the fossil. In
P. paniscus, the buccal cusps are
even more peripherally located than they are in the Ngorora tooth. Another
difference between the Ngorora tooth and
P. paniscus lies in the height
of the entoconid, which is taller and more trenchant in
P. paniscus. The
anterior fovea is buccolingually narrower in the fossil than in
Pan.
However, these are relatively minor differences.
The Ngorora molar resembles lower molars of
Pan troglodytes from
Mahale, Tanzania (
Figure 1C) more closely than it does those
of
P. paniscus. This is mainly because the buccal cusps are slightly more
internally positioned in the common chimpanzee, and they thus have moderate
buccal flare of the cusp walls. A minor difference is the extent of the mesial
fovea, which is greater in
P. troglodytes than it is in the Ngorora
specimen, and most chimpanzee molars do not have any trace of a buccal
cingulum.
Lower molars of the European genus
Dryopithecus (
Figure 1D–F) have peripheralized cusps, and broad but shallow
occlusal basins (
Begun, 2002). They also possess slight buccal
cingula and some specimens have a small accessory cusplet separating the
metaconid and entoconid in the lingual notch. The most significant differences
between the Kenyan and European specimens are the shallower occlusal basins and
the less buccolingually compressed lingual cusps that occur in
Dryopithecus. In particular, the Ngorora tooth resembles some of the central
European
D. brancoi,
especially specimens such as those from
Salmendingen, Trochtelfingen, and Ebingen (
Figure 1D–F). It
is not impossible that the Ngorora species is related to the genus
Dryopithecus.
Comparison of the Ngorora tooth (
Figure 1A) with lower
molars of African fossil hominoids reveals that it does not belong to any of the
described Miocene forms, none of which possess the large occlusal basin and
peripheralized cusps that distinguish this tooth. The only specimen with which
it accords in morphology is the Ngorora upper molar (KNM-BN 1378), which has
wide occlusal fovea and a large protocone, but this tooth is appreciably larger
than what would be expected to occlude with Bar 91’99.
Until now, no fossil chimpanzees and gorillas have been reported and there
has thus been a lack of evidence to test the molecular clock estimates of the
dichotomy between African apes and hominids. If the derived characters of the
Ngorora molar represent homologies shared with chimpanzees, then it would
indicate that the
Pan clade has its roots in the latter part of the
Middle Miocene, sometime prior to 12.5 Ma.
The dichotomy between chimpanzees and humans is usually estimated by
molecular biologists to be more recent than 6 Ma (
Gagneux et al.,
1999;
Stauffer et al., 2001) and the split between
chimpanzees and gorillas has been estimated at 8–9 Ma by
Wrangham
and Pilbeam (2001) and 7.7 Ma by
Gagneux et al. (1999).
The only molecular biologists who have proposed an earlier age for African ape
origins are Arnason and co-workers (
Arnason et al.,1996,
1998,
2000;
Janke and Arnason,
2002), but their results are usually considered suspect by others who appear
to favor appreciably later divergence times (
Bailey et al.,
1992;
Adachi and Hasegawa, 1995;
Gagneux et
al., 1999;
Stauffer et al., 2001;
Pilbeam,
2002).
The Ngorora specimen thus runs counter to the recent ideas of
Pilbeam (1996) who wrote that “the common ancestor of humans and
chimpanzees was probably chimpanzee-like, a knuckle-walker with small
thin-enamelled cheek teeth,” and
Wrangham and Pilbeam (2001)
who postulated that the “6 mybp ancestor…would have been thin-enam-elled,
knuckle-walking, and females would have had black body coats.” It is already
known that 6 Ma hominids such as
Orrorin tugenensis had thick-enamelled
molars with restricted occlusal basins and were fully bipedal (
Senut et al., 2001;
Pickford et al., 2002).
Instead the Ngorora fossil ape tooth accords with the scenario published by
Arnason et al. (1996,
1998,
2000) based on molecular evidence, of an early divergence (ca.
13.5 Ma) between
Pan and
Homo.
Description
The Kapsomin tooth is a partial left upper molar (
Figure
2A–D). It lacks the roots but the cervical line is partly preserved,
allowing estimation of cusp height. Also preserved are the entire paracone and
metacone, part of the hypocone and the distobuccal extremity of the protocone
(the crista obliqua). The distal fovea is complete. The tooth is 14 mm
mesiodistal. It is low crowned (7.3 mm from cervix to tip of metacone) and the
cusps are endowed with low but broad wrinkles.
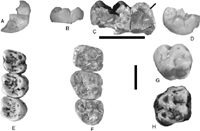
View Details |
Figure 2.
Kapsomin upper
molar and comparisons. (A–D) Bar 1757’02, Kapsomin large ape, cast of left upper
molar; (A) occlusal; (B) buccal; (C) enlarged internal view to show dentine
enamel junction and measuring point (oblique black line) for enamel thickness
determination; (D) oblique view. (E) Pan troglodytes upper M1/–M3/,
occlusal view. (F) Orrorin tugenensis upper molar row, occlusal view. NB
The toothrow of O. tugenensis comprises two teeth (M1/ and M2/) found
after the description of the M3/ (Senut et al., 2001).
Concordant interstitial facets reveal that the three teeth belong to a single
individual. (G) Gorilla gorilla M2/, oblique view. (H) G. gorilla
upper M2/, occlusal view. |
The paracone and metacone are separated from each other by a narrow slit-like
buccal groove (
Figure 2B). The part of the trigon basin
preserved is relatively large and open, the main cusps being peripherally
positioned and the cusps not bulbous. The mesial fovea is not preserved. Two low
crests descend from the apex of the paracone towards the midline of the crown
(i.e. towards the mesial fovea). The metacone sends a crest lingually and
slightly anteriorly towards the protocone, from which it is separated by a
groove. The metacone has another, smaller crest that descends obliquely distally
directly towards the hypocone, bifurcating on its way down the cusp. The
anterior branch of the crest forms a low wall between the trigon basin and the
distal fovea. The distal cingulum reaches from the base of the metacone to the
hypocone and walls off the distal fovea on the distal aspect of the tooth. The
metacone and hypocone are spaced far apart, and as a consequence, the distal
fovea is buccolingually wide and slightly obliquely oriented with the buccal end
more mesial than the lingual one. The apex of the hypocone is missing so it is
not possible to provide detailed measurements of the distance between its apex
and those of the paracone and metacone. We estimate the distance between the
tips of the hypocone and metacone at 7.5 mm. The distance between the tips of
the paracone and metacone is 6 mm. The distal margin of the crown is not
markedly curved, suggesting that it is not a third upper molar, but more likely
a second or first. The surface of the enamel is wrinkled but not as heavily as
is usually the case in chimpanzee molars. Enamel thickness measured radially (
Schwartz, 2000) 2 mm below the tip of the preserved part of the
hypocone is 1.6–1.7 mm (line in
Figure 2C), but this figure
is likely to be an overestimate of the true thickness, as it is taken slightly
obliquely due to the orientation of the fractured surface of the cusp.
Comparisons with Orrorin tugenensis
and Pliocene hominids
Bar 1757’02 is highly divergent from the teeth in the upper tooth row of
Orrorin (
Figure 2F), not only in its greater
dimensions, but also in its morphology. The trigon basin and distal fovea in the
upper molars of
Orrorin are restricted in size; the buccal cusps are more
flared from apex to cervix, the buccal slit is non-existent, the main cusps are
lower crowned, the dentine penetrance is low, and the enamel is less wrinkled.
The Kapsomin ape tooth is closer in size and some aspects of morphology to upper
molars of
Australopithecus afarensis and
Praeanthropus africanus.
However, the higher and less-inflated cusps, the steep, flat, and unflared
buccal surface, thinner enamel, and the greater dentine penetrance suggest that
Bar 1757’02 is not from an australopithecine.
Comparisons with Gorilla gorilla
Upper molars of gorillas are generally longer than Bar 1757’02 but there are
small individuals that overlap in size; upper M1/s of
Gorilla gorilla
range in mesiodistal length from 12.7 to 16.7 mm, M2/s from 13.4 to 17.9 mm, and
M3/s from 12.0 to 17.0 mm (
Pilbeam, 1969).
The crown morphology of Bar 1757’02 is similar in several respects to that of
molars of
Gorilla gorilla (
Figure 2G, H). In
particular the mesio-distally short but bucco-lingually wide distal fovea of the
Lukeino tooth resembles that of
Gorilla as does its oblique orientation.
Another resemblance lies in the degree and kind of enamel wrinkling and the high
dentine penetrance. In
G. gorilla enamel is generally considered to be
thin (ranging from 0.98 to 1.63 mm on the protocone and between 0.7 and 1.33 mm
on the paracone:
Schwartz, 2000), and in Bar 1757’02 it is
1.6–1.7 mm on the hypocone, which falls slightly above the range of variation of
Gorilla. This measure was taken on a slightly oblique natural section of
the tooth (
Figure 2C), and the radial thickness would be
slightly less than this figure.
Comparisons with Pan
Bar 1757’02 differs markedly from molars of
P. troglodytes and
P.
paniscus, not only in size, but also in morphology (
Figure
2E). M1/s and M2/s of
P. troglodytes range in mesiodistal length from
9 to 12 mm, and M3/s from 8.2 to 10.6 mm (
Pilbeam, 1969). The
trigon basins in molars of
Pan are extensive and the crests separating
the basin from the mesial and distal foveae are quite low. In any case, the
distal fovea in
Pan is reduced in buccolingual extent. A further
difference is the greater buccolingual compression of the apices of the paracone
and metacone in
Pan.
Comparisons with Sahelanthropus tchadensis
Bar 1757’02 is larger than the upper molars in
Sahelanthropus tchadensis
(
Brunet et al., 2002) (mesio-distal length is 14 mm in Bar
1757’02, compared to 10.9–11.5 mm in M1/, 13 mm in M2/, and 10.7–10.8 mm in M3/
of
Sahelanthropus).
Brunet et al. (2002) describe the
molars as having “low rounded cusps…,” in which case they are different from the
Kapsomin tooth. The enamel in the upper molars of
Sahelanthropus has been
reported to be 1.71 mm thick in the paracone of M3/ and 1.79 mm at the hypocone
of M2/, which suggests that enamel in
Sahelanthropus is approximately the
same as in the Kapsomin ape in which it is 1.6–1.7 mm on the hypocone. The
enamel thickness of
Orrorin was originally (
Senut et al.,
2001) said to be 3.1 mm on the protoconid of m/2 but this was a
typographical error that unfortunately carried through into both translated
versions of the text. The thickness measured radially near the tip of the
protoconid on the original fossil was 2.1 mm. The enamel thickness decreases
towards the cervix such that it is about 1.7 mm thick about 2 mm below the apex
of the cusp. Further study with a scanner is planned.
Comparisons with other Miocene hominoids
Bar 1757’02 differs in various features from most of the known medium and
large hominoids from the Miocene of Africa. Molar morphology in
Kenyapithecus,
Nacholapithecus,
Otavipithecus, and
Afropithecus is quite different, these taxa possessing non-peripheralized
bulbous main cusps and restricted occlusal basins (
Conroy et al.,
1992;
Ward and Duren, 2002). In
Proconsul and
Ugandapithecus the cusps are not peripheralized (
Harrison,
2002;
Senut et al., 2000). The cusps in the upper molars
of
Samburupithecus are inflated, and the occlusal basins are extremely
restricted (
Pickford and Ishida, 1998), which makes them very
divergent from the Kapsomin tooth.
Ouranopithecus and
Ankarapithecus from European Late Miocene
deposits have thick-enamelled molars with restricted occlusal basins and low
dentine penetrance, interpreted by
de Bonis et al. (1981) as
features shared with Hominidae, from which we infer that the genus is markedly
different from the Kapsomin ape.
Discussion
It seems clear that the fragment of upper molar from Kapsomin (Bar 1757’02)
represents a species distinct from
O. tugenensis. Apart from its greater
dimensions, it has morphology that is different from the bunodont crown with
inflated main cusps and restricted trigon basin and foveae of the latter taxon.
Whereas the molars of
Orrorin recall those of later hominids
(
Australopithecus,
Praeanthropus, and even
Homo) in overall
crown shape, bunodonty, cusp inflation, and basin restriction, Bar 1757’02
stands out as anomalous, with its more peripheralized cusps, widely separated
metacone and hypocone, wide, obliquely oriented distal fovea, vertical and flat
buccal surface, high dentine penetrance, and thinner enamel with light
wrinkling. The tooth has high dentine penetrance as shown by the fact that if it
were worn to the same level as the M2/ in the
Orrorin tooth row, which
shows no dentine exposure, the Kapsomin ape tooth would have a large dentine
exposure (the shadow at the apex of the protocone of the M2/ of
Orrorin
in
Figure 2F is a small patch of chemical etching
floored by enamel and is not an exposure of dentine). The morphology of the
dentine-enamel junction in the Kapsomin tooth is best appreciated by examining
the broken inner surface of the Kapsomin tooth where it is clearly visible,
showing the dentine penetrating high into the hypocone (
Figure
2C). In most of these features the tooth is closest to
G. gorilla,
yet it is by no means a perfect fit with this species.
Metrically, the Kapsomin tooth is appreciably larger than any of the
Orrorin molars, but it falls within the range of metric variation of the
gorilla. It also falls within the range of variation of Pliocene fossil
hominids, including
Ardipithecus,
Australopithecus, and
Praeanthropus. Its relationships to
Sahelanthropus are not clear
as published photographs of the latter do not reveal enough detail about crown
morphology, but descriptions of the fossil suggest major morphological
differences.
We conclude that Bar 1757’02 reveals the presence of a second hominoid in the
Lukeino Formation, Kenya. Its affinities appear to lie with gorillas rather than
hominids. Its dimensions fall within the range of variation of gorilla upper
molars and well above that of chimpanzees, and we discount any close
relationship with
Pan, which, apart from being smaller and having
peripheralized main cusps, has quite different molar crown morphology.
Bar 1001’00 is a right upper central incisor that has been abraded mesially
and distally (
Figure 3B). The preserved part of the crown is
relatively low compared to root length. In lateral view the labial and lingual
surfaces form a wedge-shape; the tooth is 8.7 mm thick labio-lingually near the
cervix and narrows rapidly incisally. It has lightly wrinkled enamel. The crown
is unlike the corresponding teeth of australopithecines and humans because there
is no fossa on the lingual side (
Figure 3A). In hominids the
crown is higher from cervix to incisal edge and the lingual surface has a large
fossa, sometimes with lingual ridges or pillars, but usually possessing a
scoop-shaped profile. In gorillas, in contrast, the upper central incisors are
often wedge-shaped, with no sign of a lingual fossa, or if one is developed it
is not very prominent. Comparison of the Kapsomin upper incisor with those of
gorillas reveals close similarities (
Figure 3C) not only in
size but also in shape and crown height relative to root length. Even though
these similarities are enhanced by the wear stage of the Kapsomin incisor and
that of the gorilla used for comparison, this is because the underlying
morphology is similar. Chimpanzee and hominid teeth worn as much as the Kapsomin
fossil do not develop a comparable wedge-shaped appearance in lateral view,
because the lingual surface of their teeth is basin-like.
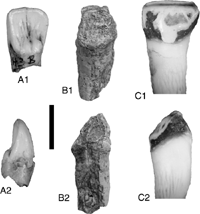
View Details |
Figure 3.
Kapsomin upper
incisor and comparisons. (A) Praeanthropus africanus cast of upper
central incisor from Laetoli; (A1) lingual view; (A2) distal view. (B) Bar
1001’01, cast of upper central incisor, Kapsomin large ape; (B1) lingual view;
(B2) distal view. (C) Gorilla gorilla upper central incisor worn to the
same level as Bar 1001’01; (C1) lingual view; (C2) distal
view. |
An anonymous referee considered that this tooth is similar to A.L. 198-17a
(
Johanson et al., 1982) and on this basis suggested that the
Kapsomin tooth may belong to an australopithecine. However, comparison of the
specimens reveals that they are divergent in morphology. The Hadar fossil has a
restricted basal lingual pillar bordered mesially and distally by hollows, as in
other australopithecine teeth, whereas Bar 1001’00 does not, its basal lingual
part being inflated right across the preserved part of the tooth, as in gorillas
with which we compared the tooth. Furthermore, the wear facet in A.L. 198-17a is
apical, flat, and at right angles to the long axis of the tooth, whereas that in
the Kapsomin tooth is steeply angled from incisal edge to cervix on the lingual
side, again as in gorillas. In apical view the Hadar tooth is a mesiodistally
elongated rectangle, slightly concave lingually with slight projection of the
basal tubercle, whereas the Kapsomin tooth is much broader labiolingually. This
is reflected in the dimensions of the teeth, the Kapsomin specimens measuring
8.7 mm labiolingually near cervix and the Hadar specimen only 7.1 mm. Larger
upper central incisors from Hadar (A.L. 200-1a, A.L. 333x-4, and A.L. 333x-20)
measure 8.5, 8.6, and 8.6 mm labiolingually, respectively.
Discussion
The upper central incisor (Bar 1001’00) was initially attributed to
O.
tugenensis (
Senut et al., 2001) because at the time of the
discovery it was assumed that only a single hominoid was represented at the
site. With the discovery of Bar 1757’02, a partial upper molar, it became clear
that a second, larger, hominoid taxon was present at Kapsomin, leading to a
re-evaluation of all the specimens from the site. Because of its dimensions
(labiolingual breadth 8.7 mm) and its somewhat gorilla-like morphology and wear
pattern, we now consider that the upper central incisor belongs to this second
hominoid rather than to
Orrorin.
Bar 2000’03 from Cheboit, Lukeino Formation, is an unworn right lower molar
lacking the roots, but preserving much of the cervical line (
Figure 4). The apices of the two lingual cusps are
buccolingually compressed and peripherally located. The tips of the protoconid
and metaconid are 5.4 mm apart and the tooth is 10.5 mm broad at this level. The
two buccal cusps are slightly in advance of the lingual ones, and there is minor
buccal flare. Because of the peripheral positions of the main cusps, the
occlusal basin is large and elongated. The mesial fovea is wide but
mesiodistally short. The hypoconulid is small and is located slightly to the
lingual side of the centre line of the tooth and in a very distal position, and
as a result the tooth has an elongated trapezoidal outline (
Figure 4). Because of this the tooth could be a lower third
molar. The distal fovea is thus small, but is not separated from the main
occlusal basin by the crests from the hypoconulid or entoconid, as these do not
reach each other. The tooth measures 12.7 mm mesiodistal by 11.1 mm
buccolingual.
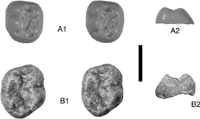
View Details |
Figure 4.
Cheboit lower molar
and comparisons. (A) KNM-LU 335, Orrorin tugenensis, cast of left m/3,
stereo occlusal view. NB KNM-LU 335 was originally interpreted to be an m/2
(Andrews in Pickford, 1975), but comparison with teeth in the holotype mandible
of O. tugenensis reveals that it is in fact an m/3. (B) Bar 2000’03,
Cheboit large ape right lower molar; (B1) stereo occlusal view; (B2) mesial
view. |
This tooth is morphologically compatible with Bar 1757’02, the upper molar
from Kapsomin. We consider it likely that the two specimens belong to a single
taxon.
This lower molar differs radically from those of
O. tugenensis of
which it is a contemporary. The occlusal outline is long, narrow, and
trapezoidal compared with a wider, more rectangular outline in
Orrorin.
The main occlusal basin is deep, wide, and long, compared with the shallow,
narrow, and short basin in
Orrorin. The distal fovea is not separated
from the main basin, whereas in
Orrorin it is. The buccal flare is not as
marked as it is in
Orrorin and the lingual cusps are more peripherally
located. The main cusps are not as inflated as those of
Orrorin,
suggesting that the tooth has thinner enamel and probably greater dentine
penetrance, but we have not yet had the opportunity to determine these
parameters. In
Orrorin, the hypoconulid is located to the buccal side of
the midline, and is thus close to the hypoconid, whereas in Bar 2000’03 it is to
the lingual side of midline, and far from the hypoconid. Indeed this cusp is
closer to the entoconid than it is to the hypoconid, the opposite of the
situation in
Orrorin. In the latter genus, there is a clear valley
buccally between the hypoconid and the entoconid, whereas in Bar 2000’02, there
is not even an indentation in the distobuccal wall of the tooth. The latter
tooth is also somewhat bigger than any of the
Orrorin lower
molars.
Four ape-like teeth from the Miocene of Kenya reveal greater similarities to
extant chimpanzee and gorilla teeth than to thick-enamelled Miocene apes and
Mio–Plio–Pleistocene to recent hominids. The specimen from Ngorora (12.5 Ma) is
similar in size and some morphological details to
Pan but also has
resemblances to the European Miocene genus
Dryopithecus, with which it
could be congeneric, whereas the Lukeino specimens (5.9 Ma), recall, but are not
identical to, the teeth of gorillas.
The morphology of the Ngorora tooth suggests that the
Dryopithecus
lineage may have evolved in Africa and then invaded Europe about 12–12.5 Ma,
rather than evolving within Europe from a thick-enamelled lineage such as
Griphopithecus (
Begun, 2002). If it is part of the
Pan clade, then it would push back the split between hominids and African
apes to the Middle Miocene. If this is so, then thick-enamelled apes such as
Kenyapithecus possibly take on a renewed significance for throwing light
on the earliest stages in the evolution of hominids, as thought by L. Leakey in
the 1960s (
Leakey, 1962,
1967,
1969,
1970) even though the supposedly hominid
features employed by Leakey in his proposals have subsequently been interpreted
as being related to sexual dimorphism and to plesiomorphic features found in
several Middle Miocene hominoids, rather than to derived morphology shared with
hominids (
Pickford, 1985). It is more parsimonious to consider
that thick-enamelled hominids descended from thick-enamelled precursors rather
than to hypothesize a thin-enamelled intermediate stage, as has apparently
become the fashion (
Wrangham and Pilbeam, 2001). What is
required is a fresh look at the problem, including the relationships between
diet on the one hand and enamel thickness and dentine penetrance on the
other.
If the Kapsomin ape teeth belong to the gorilla clade, then they would
indicate that by about 6 Ma the lineage was a separate entity from the
Pan +
Homo clade. Taken together, the Ngorora and Kapsomin ape
teeth, and those of the early bipedal hominid
Orrorin, plead for
considerably earlier split times between the gorilla, chimpanzee, and hominid
clades than most molecular biologists have considered possible for the past
three decades (
Gagneux et al., 1999;
Stauffer et
al., 2001) but more in accord with the results of Arnason and his colleagues
(
Arnason et al., 1996,
1998,
2000;
Janke and Arnason, 2002).
The four teeth from Baringo district, Kenya, described here reveal the
presence of ape-like hominoids in East Africa during the latter part of the
Middle Miocene and the Late Miocene. They thus refute the statement by
Begun (2002) that “In actual fact, none of the many late Miocene
African fossil localities has any hominoids….” When we add them to
Samburupithecus from the Late Miocene of Samburu Hills, Kenya (9.5 Ma)
(
Ishida and Pickford, 1997),
Orrorin from Lukeino,
Kenya (6–5.7 Ma) (
Senut et al., 2001),
Sahelanthropus
from Toros-Menalla, Chad (ca. 7–6 Ma) (
Brunet et al., 2002),
and
Ardipithecus from Ethiopia (
White et al., 1994,
1995), it is clear that Late Miocene Africa was not devoid of
hominoids until they reintroduced themselves from Europe (
Begun,
2002). Rather, it is more likely that chimp-sized
Dryopithecus was
originally an African lineage that invaded western Europe about 12.5–12 Ma, and
while the evidence is scant, some of the large gorilla-sized hominoids from the
Late Miocene of Greece and Turkey could also be of African origin rather than
autochthonously evolved descendents of
Dryopithecus or
Sivapithecus as envisaged by
Begun (2002). Despite the
relative poverty of the African fossil record, the new discoveries reveal that
hominoids were more diverse in the Late Miocene of Africa than they were in
Europe (five genera now known in Africa compared to three or perhaps four in
Europe).
We thank members of the Kenya Palaeontology Expedition for their help in the
field, in particular Mr Kiptalam Cheboi. Research permission was accorded by the
Kenya Ministry of Education, Science and Technology. Funds were provided by the
Collège de France (Professor Y. Coppens), the Département Histoire de la Terre
(Professor Ph. Taquet), the French Ministry of Foreign Affairs (Commission des
Fouilles), and the CNRS (Projet PICS). We are particularly keen to thank the
Community Museums of Kenya (Mr E. Gitonga) for their help and cooperation and
Professor H. Ishida for inviting MP to spend time in his laboratory as visiting
professor at Kyoto University where he was able to examine the extensive cast
collection of extant and fossil hominoids that has been amassed. We also thank
the Primate Research Institute, Inuyama, Japan and Yutaka Kunimatsu for
discussions.
Adachi J. and Hasegawa M. (1995) Improved
dating of the human-chimpanzee separation in the mitochondrial DNA tree:
heterogeneity among amino acid sites. Journal of Molecular Evolution, 40:
622–628. |
Arnason U., Gullberg A., Janke A., and
Xiufeng Xu (1996) Pattern and timing of evolutionary divergences among hominoids
based on analyses of complete mtDNAs. Journal of Molecular Evolution, 43:
650–651. |
Arnason U., Gullberg A., and Janke A.
(1998) Molecular timing of Primate divergences as estimated by two nonprimate
calibration points. Journal of Molecular Evolution, 47: 718–727. |
Arnason U., Gullberg A., Schweitzer
Burguette A., and Janke A. (2000) Molecular estimates of Primate divergences and
new hypotheses for Primate dispersals and the origin of modern humans.
Hereditas, 133:
217–228. |
Bailey W.J., Hayasaka K., Skinner C.G.,
Kehoe S., Sieu L.C., Slightom J.L., and Goodman M. (1992) Reexamination of the
African hominoid trichotomy with additional sequences from the primate β-globin
gene cluster. Molecular Phylogenetics and Evolution, 1: 97–135. |
Begun D. (2002)
European hominoids. In: Hartwig W.C. (ed.), The Primate Fossil Record. Cambridge
University Press, Cambridge, pp. 339–368. |
Brunet M., Guy F., Pilbeam D., Mackaye
H., Likius A., Ahounta D., Beauvilain A., Blondel C., Bocherens H., Boisserie
J.-R., de Bonis L., Coppens Y., Dejax J., Denys C., Duringer P., Eisenmann V.,
Fanone G., Fronty P., Geraads D., Lehmann T., Lihoreau F., Louchart A., Mahamat
A., Merceron G., Mouchelin G., Otero O., Campomanes P., Ponce de Leon M., Rage
J.-C., Sapanet M., Schuster M., Sudre J., Tassy P., Valentin X., Vignaud P.,
Viriot L., Zazzo A., and Zollikofer C. (2002) A new hominid from the Upper
Miocene of Chad, Central Africa. Nature, 418:
145–151. |
Conroy G., Pickford M., Senut B., Van
Couvering J., and Mein P. (1992) Otavipithecus namibiensis, first Miocene
hominoid from Southern Africa (Berg Aukas, Namibia). Nature, 356 : 144–148. |
de Bonis L., Johanson D., Melentis J.,
and White T. (1981) Variations métriques de la denture chez les Hominidés
primitifs: comparaison entre Australopithecus afarensis et
Ouranopithecus macedoniensis. Comptes Rendus des Séances de l’Académie
des Sciences, Série 2, 292: 373–376. |
Gagneux P., Wills C., Gerloff U., Tautz
D., Morin P., Boesch C., Fruth B., Hohmann G., Ryder O., and Woodruff D. (1999)
Mitochondrial sequences show diverse evolutionary histories of African
hominoids. Proceedings of the National Academy of Sciences of the United States
of America, 96:
5077–5082. |
Harrison T.
(2002) Late Oligocene to Middle Miocene catarrhines from Afro-Arabia. In:
Hartwig, W.C. (ed.), The Primate Fossil Record. Cambridge University Press,
Cambridge, pp.
311–333. |
Ishida H. and Pickford M. (1997) A new
late Miocene hominoid from Kenya: Samburupithecus kiptalami gen. et sp.
nov. Comptes Rendus de l’Académie des Sciences de Paris, Series 2A, 325:
823–829. |
Janke A. and
Arnason U. (2002) Primate divergence times. In: Galdikas B., Briggs N., Sheeran
L., Shapiro G., and Goodall J. (eds.), All Apes Great and Small. Volume 1:
African Apes. Kluwer Academic/Plenum Publishers, New York, pp. 18–25. |
Johanson D., White T., and Coppens Y.
(1982) Dental remains from the Hadar Formation, Ethiopia: 1974–1977 collections.
American Journal of Physical Anthropology, 57:
545–603. |
Leakey L.S.B. (1962) A new lower
Pliocene fossil primate from Kenya. Annals and Magazine of Natural History,
Series 13, 4:
689–696. |
Leakey L.S.B. (1967) An early Miocene
member of Hominidae. Nature, 213: 155–163. |
Leakey L.S.B. (1969) Fort Ternan
hominid. Nature, 222:
1202. |
Leakey L.S.B. (1970) The relationships
of African apes, man and Old World monkeys. Proceedings of the National Academy
of Sciences of the United States of America, 67: 746–748. |
Pickford M. (1975) Late Miocene
sediments and fossils from the Northern Kenya Rift Valley. Nature, 256: 279–284. |
Pickford M. (1985) A new look at
Kenyapithecus based on recent collections from Western Kenya. Journal of
Human Evolution, 14: 113–143. |
Pickford M. and Ishida H. (1998)
Interpretation of Samburupithecus, an upper Miocene hominoid from Kenya.
Comptes Rendus de l’Académie des Sciences de Paris, Série 2A, 326: 299–306. |
Pickford M., Senut B., Gommery D., and
Treil J. (2002) Bipedalism in Orrorin tugenensis revealed by its femora.
Comptes rendus Palevol, 1: 191–203. |
Pilbeam D.R. (1969) Tertiary Pongidae of
East Africa: evolutionary relationships and taxonomy. Bulletin of the Peabody
Museum of Natural History, 31: 1–185. |
Pilbeam D. (1996) Genetic and
morphological records of the Hominoidea and hominid origins: a synthesis.
Molecular Phylogenetics and Evolution, 5: 155–168. |
Pilbeam D.
(2002) Perspectives on the Miocene Hominoidea. In: Hartwig W.C. (ed.), The
Primate Fossil Record. Cambridge University Press, Cambridge, pp. 303–310. |
Schwartz G.T. (2000) Taxonomic and
functional aspects of the patterning of enamel thickness distribution in extant
large-bodied hominoids. American Journal of Physical Anthropology, 111: 221–244. |
Senut B. (1998)
Les grands singes fossiles et l’origine des hominidés: mythes et réalités.
Primatologie, 1:
93–134. |
Senut B., Pickford M., Gommery D., and
Kunimatsu Y. (2000) Un nouveau genre d’hominoïde du Miocène inférieur d’Afrique
orientale: Ugandapithecus major (Le Gros Clark and Leakey, 1950). Comptes
Rendus de l’Académie des Sciences de Paris, Série 2A, 331: 227–233. |
Senut B., Pickford M., Gommery D., Mein
P., Cheboi K., and Coppens Y. (2001) First hominid from the Miocene (Lukeino
Formation, Kenya). Comptes Rendus de l’Académie des Sciences de Paris, Série 2A,
332: 137–144. |
Stauffer R. L., Walker A., Ryder O.,
Lyons-Wailer M., and Hedges S. (2001) Human and ape molecular clocks and
constraints on paleontological hypotheses. Journal of Heredity, 92: 469–474. |
Ward S. and
Duren D. (2002) Middle and Late Miocene African hominoids. In: Hartwig W.C.
(ed.), The Primate Fossil Record. Cambridge University Press, Cambridge, pp.
385–397. |
White T., Suwa G., and Asfaw B. (1994)
Australopithecus ramidus, a new species of early hominid from Aramis,
Ethiopia. Nature, 371: 306–312. |
White T., Suwa G., and Asfaw B. (1995)
Australopithecus ramidus, a new species of early hominid from Aramis,
Ethiopia. Nature, 375:
88. |
Wrangham R. and
Pilbeam D. (2001) African apes as time machines. In: Galdikas B., Briggs N.,
Sheeran L., Shapiro G., and J. Goodall J. (eds), All Apes Great and Small.
Volume 1: African Apes. Kluwer Academic/Plenum Publishers, New York, pp. 5–17. |
|
|
Copyright © 2005 The Anthropological Society of
Nippon
No comments:
Post a Comment
This blog does NOT allow anonymous comments. All comments are moderated to filter out abusive and vulgar language and any posts indulging in abusive and insulting language shall be deleted without any further discussion.